Welcome to ElectricalEngineeringAssignmentHelp.com, your premier destination for mastering the intricacies of electrical engineering assignments! In this comprehensive guide, we'll delve into essential concepts and problem-solving techniques to empower you in conquering Electromagnetism assignments effectively. Whether you're navigating power conversion devices or grappling with complex energy storage calculations, understanding the fundamental principles and methodologies is paramount. So, let's embark on this enlightening journey together! Electrical engineering stands as a cornerstone of modern technological advancement, encompassing a vast array of disciplines from power generation to microelectronics. At its core lies the manipulation and control of electrical energy, a fundamental force that drives innovation across industries. For students and practitioners alike, navigating the complexities of electrical engineering assignments demands both a solid theoretical foundation and practical problem-solving skills. This is where ElectricalEngineeringAssignmentHelp.com steps in — as your dedicated resource to unravel these complexities and foster deeper understanding. The field of electromagnetic energy, central to electrical engineering, spans a broad spectrum of applications. From the generation and distribution of electrical power to the design of integrated circuits, electromagnetic principles underpin the functioning of countless devices and systems. Mastering these concepts begins with a clear grasp of electromagnetic theory, which elucidates the interactions between electric and magnetic fields. At ElectricalEngineeringAssignmentHelp.com, we break down these theoretical constructs into digestible segments, empowering learners to apply them confidently in assignments and real-world scenarios.
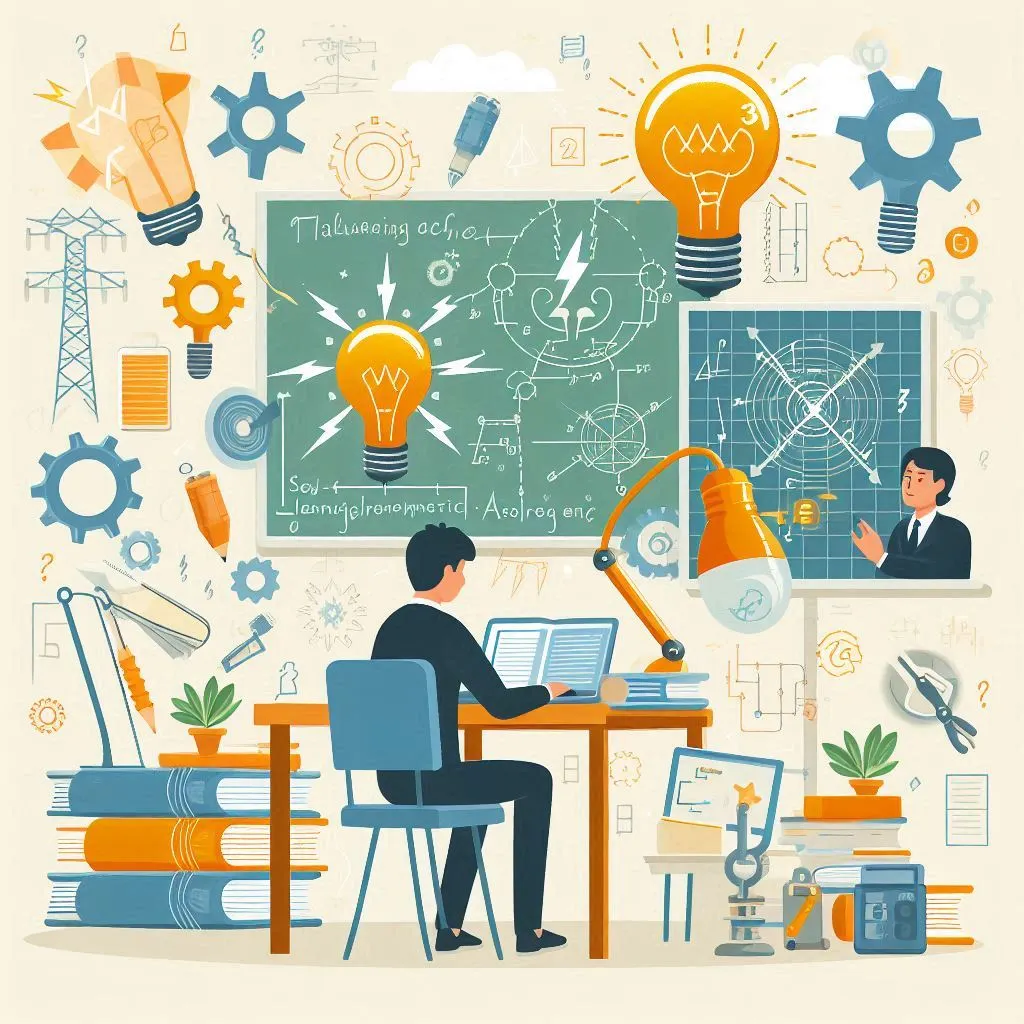
Power conversion devices represent a pivotal area within electromagnetic energy studies. These devices, ranging from transformers to inverters, facilitate the efficient conversion of electrical energy between different forms and levels. Understanding their operation involves delving into circuit theory, semiconductor physics, and control systems — disciplines that converge to ensure optimal energy transfer and utilization. Our guides at ElectricalEngineeringAssignmentHelp.com elucidate the underlying principles governing these devices, equipping learners to analyze their performance and design considerations comprehensively. Energy storage calculations pose another significant challenge in electrical engineering assignments. Whether calculating the capacitance of a capacitor bank or determining the charge-discharge characteristics of a battery, accurate energy storage analysis hinges on a firm grasp of electrical circuits and energy conservation principles. Here, our tailored approach at ElectricalEngineeringAssignmentHelp.com emphasizes practical problem-solving strategies alongside theoretical frameworks, bridging the gap between classroom learning and application. Navigating through these complexities requires more than just theoretical knowledge; it demands hands-on experience and analytical acumen. At ElectricalEngineeringAssignmentHelp.com, we integrate practical examples and case studies into our tutorials, offering learners a holistic learning experience. By simulating real-world scenarios, we enable students to translate abstract concepts into actionable solutions, thereby enhancing their problem-solving capabilities.
Understanding Power Conversion Devices
Power conversion devices are the bedrock of modern electrical engineering, responsible for transforming energy between different forms to meet diverse technological needs. Each device serves a unique purpose, leveraging fundamental principles of physics to enable efficient energy conversion. Here, we delve into the workings and significance of several key power conversion devices:
1. DC Motor
At the heart of many mechanical systems lies the DC motor, a prime example of converting electrical energy into mechanical motion. Operating on the principles of electromagnetic induction, DC motors rely on the interaction between magnetic fields and current-carrying conductors to generate rotational force. This transformation process hinges on specific input and output variables:
Input Through Variable: Electrical current (I)
Input Across Variable: Voltage (V)
Output Through Variable: Torque (τ)
Output Across Variable: Angular velocity (ω)
Understanding these variables allows engineers to optimize the motor's performance and efficiency. By controlling current and voltage, they can regulate torque and speed, crucial for applications ranging from industrial machinery to robotics. The versatility of DC motors underscores their ubiquitous presence in modern technology, driving innovations in automation and beyond.
2. Generator
In contrast to DC motors, generators excel in converting mechanical energy into electrical energy. Found in renewable energy systems harnessing wind, water, or steam power, generators play a pivotal role in sustainable energy production. They operate on principles similar to motors but in reverse, transforming rotational mechanical energy into electrical power. This capability is essential for renewable energy sources where mechanical motion, derived from natural forces, is converted into usable electricity.
3. Transformer
Among the silent heroes of electrical engineering is the transformer, a static device indispensable for efficient power transmission and distribution. Its primary function lies in altering voltage levels as electricity travels from power stations to end-users. Transformers achieve this through electromagnetic induction between primary and secondary coils, efficiently stepping voltage up for transmission or down for distribution. This process minimizes energy loss and ensures safe electricity delivery across vast distances, underpinning the reliability of modern electrical grids.
4. Thermoelectric Generator
The thermoelectric generator represents a cutting-edge application of thermoelectricity, harnessing temperature differentials to produce electrical power. This technology finds diverse applications, from recovering waste heat in industrial processes to powering remote sensors in space exploration. Thermoelectric generators offer a clean and efficient energy solution, converting heat directly into electricity without moving parts or emissions. As research advances, their potential grows for broader adoption in energy-efficient systems and renewable energy technologies.
Unveiling the Mysteries of Energy Storage and Conversion Calculations
Energy storage and conversion calculations are pivotal in understanding the functionality and capabilities of modern power systems, especially in vehicles like the Toyota Prius, where efficient energy management is essential. In this exploration, we delve into the methodologies and implications of energy storage, starting with the specifics of the Prius's battery system.
Problem: Energy Stored in a Toyota Prius
The Toyota Prius, renowned for its hybrid electric drivetrain, utilizes a battery pack as a key component of its energy storage architecture. To comprehensively analyze its energy storage capacity, we begin by examining the provided specifications: an output voltage of 201.6 volts and a total capacity of 1310 watt-hours (Wh). Additionally, the mass of the battery itself is documented at 45 kilograms, contributing to the overall mass of the vehicle, which stands at 1328 kilograms when fully equipped with the battery:
Specs:
Output Voltage: 201.6 V
Capacity: 1310 Wh
Mass of Battery: 45 kg
Mass of Car (with battery): 1328 kg
Calculations:
Energy Stored in the Battery:
The energy stored in the Prius's battery can be determined using the formula:
E=VxQ
where EEE is the energy stored, VVV is the voltage, and QQQ is the capacity in watt-hours. Substituting the given values:
E=201.6 V x 1310 Wh
Calculating this gives us the total energy stored in the battery, which serves as the primary reservoir of electrical energy for the vehicle's propulsion and auxiliary systems.
Height the Car Would Rise:
Next, we explore the hypothetical scenario of using the stored energy to lift the entire mass of the Prius vertically. This calculation involves understanding the conversion of stored electrical energy into gravitational potential energy:
Epotential=m×g×h
where m is the mass of the car including the battery, g is the acceleration due to gravity, and h is the height the car would ascend.
By equating the stored energy to the potential energy, we derive the height h the car would reach if all stored energy were converted into lifting the car against gravity.
Speed of the Car in Free Fall:
Exploring the dynamics further, we consider the scenario where the car descends under the influence of gravity alone. Here, the potential energy converts into kinetic energy:
Ekinetic=1/2mv2
where v is the speed of the car during free fall.
The relationship between potential and kinetic energy elucidates the interplay between stored energy and dynamic motion, highlighting the energy conversion processes within the vehicle's operational dynamics.
Mass of Nickel Ions:
Finally, delving into the chemistry of the battery, we scrutinize the mass of nickel ions involved in the electrochemical reactions responsible for energy storage. This inquiry into molecular dimensions underscores the fundamental role of materials science in enhancing energy storage efficiency and longevity in hybrid electric vehicles. Through these calculations and explorations, the complexities of energy storage and conversion in the Toyota Prius are systematically unraveled. From fundamental electrochemical principles governing energy density to practical applications in vehicle dynamics, each facet contributes to a holistic understanding of modern energy technologies.
Problem-Solving Techniques: A Blueprint for Success
In the realm of electromagnetic energy assignments, where complexities abound, the ability to navigate through challenges effectively hinges on a well-developed set of problem-solving techniques. This blueprint for success outlines a systematic approach that not only enhances understanding but also fosters proficiency in tackling intricate problems.
Break Down the Problem:
The first cornerstone of effective problem-solving in electromagnetic assignments is the art of breaking down complex problems into smaller, more manageable components. This initial step is crucial as it allows for a clear understanding of the task at hand. By identifying key variables and relationships within the problem statement, one can begin to delineate the pathways towards a solution. For instance, when confronted with a problem involving electromagnetic waves, breaking down the problem might involve separating the wave propagation characteristics from the boundary conditions or material properties. This decomposition not only simplifies the problem but also provides a structured approach to finding a solution.
Utilize Consistent Units:
Consistency in units is another fundamental aspect of problem-solving that cannot be overstated. In the realm of electromagnetics, where calculations often involve physical quantities like electric field strength (measured in volts per meter) or magnetic flux density (measured in teslas), using consistent units throughout the analysis is paramount. This practice not only reduces the risk of computational errors but also aids in maintaining conceptual clarity. For instance, converting all quantities to SI units ensures that calculations involving different physical parameters remain coherent and aligned with accepted standards. By adhering to consistent units, one mitigates the potential for misconceptions and ensures that the derived solutions accurately reflect the underlying physical reality.
Apply Fundamental Principles:
Grounding problem-solving efforts in fundamental principles forms the bedrock upon which accurate analyses are built. In electromagnetic assignments, principles of conservation (such as conservation of energy or momentum) and equilibrium (such as static equilibrium in electrostatics problems) play pivotal roles. By understanding and applying these principles, one not only validates the approach but also ensures the integrity of the solution process. For example, when analyzing the behavior of electromagnetic fields in a conducting medium, applying Maxwell's equations alongside principles of charge conservation helps in deriving meaningful conclusions about the distribution of electric and magnetic fields..
Verify and Validate:
The scrutiny of every step in the problem-solving process is essential for ensuring the accuracy and reliability of the final solution. Verification involves double-checking calculations, ensuring that mathematical operations are performed correctly and consistently. Validation, on the other hand, entails comparing the obtained results against physical intuition and expected outcomes. In the context of electromagnetic assignments, this might involve verifying numerical solutions against analytical predictions or experimental data. For instance, when calculating the resonance frequency of an electromagnetic waveguide, validating the computed values against theoretical expectations ensures that the solution aligns with established electromagnetic theory.
Practice, Practice, Practice:
Mastery of problem-solving techniques in electromagnetics, as in any discipline, is a product of consistent practice. Regular engagement with problems of varying complexity not only reinforces theoretical knowledge but also enhances problem-solving skills. Through repeated exposure to different types of electromagnetic assignments—ranging from straightforward applications of Ohm's law to intricate calculations involving antenna arrays—one develops a repertoire of strategies and approaches. This accumulation of experience cultivates confidence in tackling novel problems and fosters a deeper understanding of electromagnetic principles. Moreover, practice instills resilience in the face of challenges, equipping individuals with the tenacity needed to persevere through difficult problem-solving scenarios.
Conclusion:
In conclusion, mastering electromagnetic energy assignments is not merely a quest for academic achievement but a journey towards engineering excellence. By understanding the fundamental principles underpinning power conversion devices, and honing your problem-solving acumen, you can navigate through the complexities of electrical engineering with confidence and proficiency. At ElectricalEngineeringAssignmentHelp.com, we're committed to empowering the electrical engineers of tomorrow, providing comprehensive guidance and support every step of the way. So, let's embark on this transformative journey together – the future of electrical engineering awaits!